Cardenas Lab
Manipulamos la luz dentro de chips que caben en la punta de un alfiler.
Bienvenidos a Cardenas Lab
Cardenas Lab es un agradable grupo de investigación que trabaja para transformar la manera en que la gente hace uso de la ciencia y la tecnología utilizando dispositivos ópticos nanométricos.
En Cardenas Lab utilizamos técnicas de micro y nanofabricación para el proceso de diseño, desarrollo, optimización y construcción de nanoestructuras en la elaboración de chips ópticos que sirvan para mejorar las computadoras, las comunicaciones y los sensores.
La nanofabricación consiste en la creación de estructuras a escala nanométrica para obtener un comportamiento preciso de transmisión o reflejo de la luz que permita ejercer control sobre la misma y en Cardenas Lab trabajamos con el material que sea más adecuado, desde los recién descubiertos materiales 2D hasta el vidrio.
Algunos de los retos a los que buscamos solución son los siguientes:
- ¿Cómo se pueden encapsular chips fotónicos que resulten accesibles para producción masiva y a bajo costo?
- ¿Qué herramientas podemos concebir y fabricar para revolucionar otros campos científicos como la neurociencia, la biología celular o la astronomía?
- ¿Cuál nueva plataforma revolucionará a la Nanofotónica?
En Cardenas Lab los estudiantes diseñan y fabrican dispositivos nanofotónicos para resolver (o dar respuesta a) algunos de los problemas (o preguntas) difíciles en la actualidad.
Misión
Preparar estudiantes para que logren sus metas profesionales en la industria o la academia.
Revolucionar la ciencia y la tecnología de campos interdisciplinarios mediante la fotónica.
Transformar el campo de la fotónica usando materiales, técnicas y dispositivos nuevos.
Nuestra Comunidad
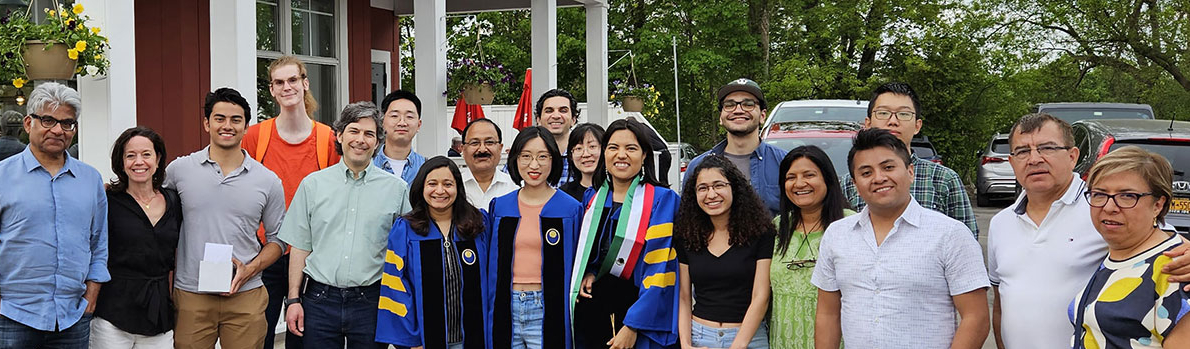
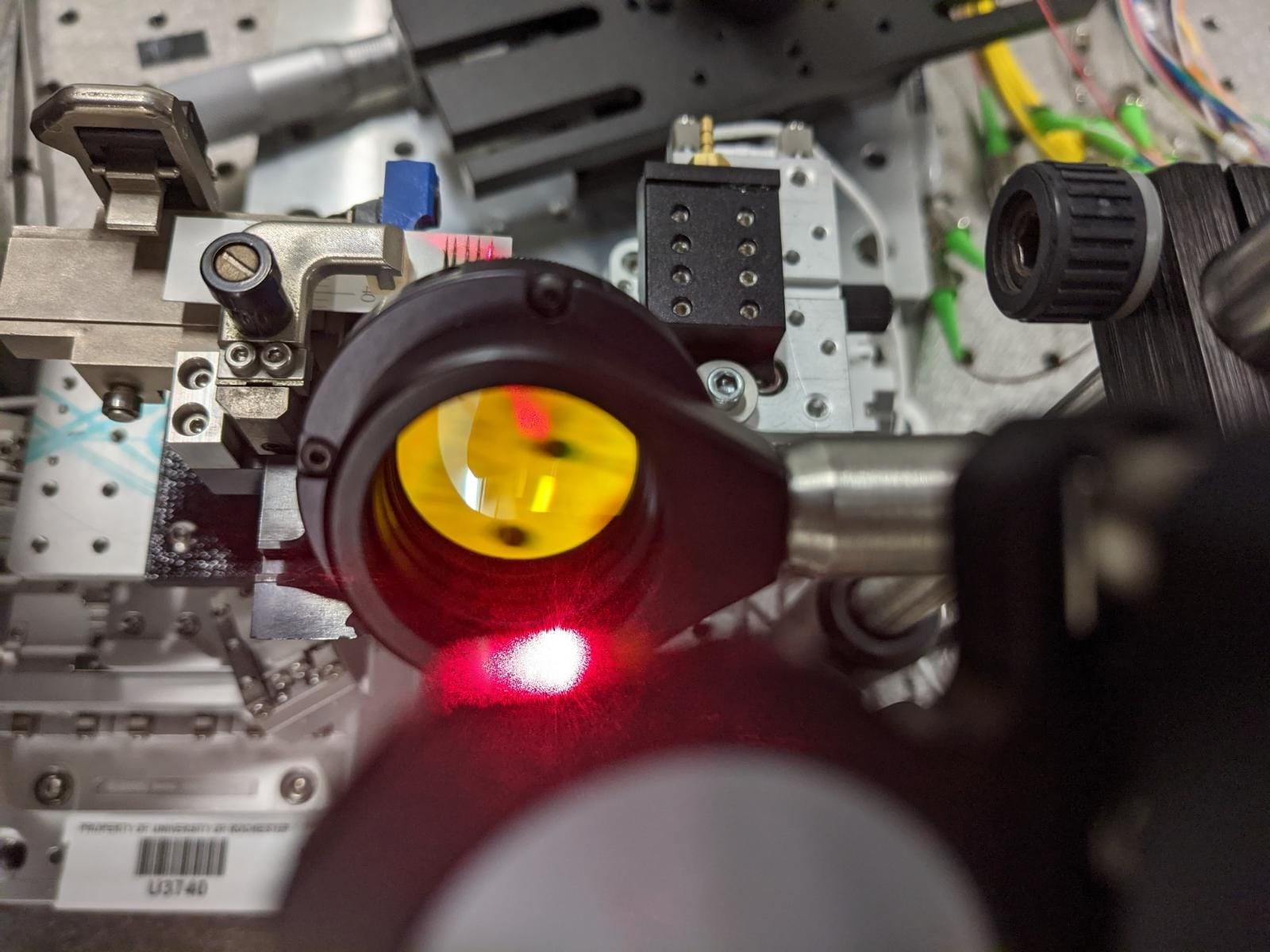
Investigación
Actualmente, las áreas de nuestra investigación son los nuevos materiales ópticos, los nuevos dispositivos y el encapsulado de dispositivos.
Nuestra Área de Trabajo
Localizada en el Instituto de Óptica de la Universidad Rochester
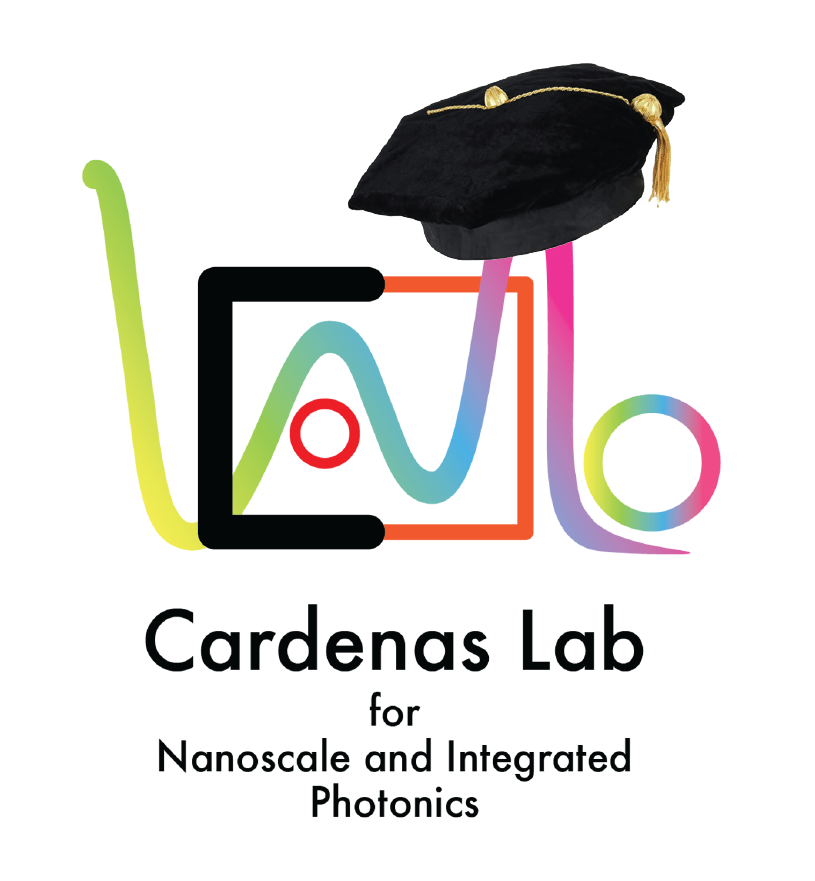
Historias de los Graduados
Los graduados del grupo nos cuentan de su tiempo como estudiantes en nuestro equipo.
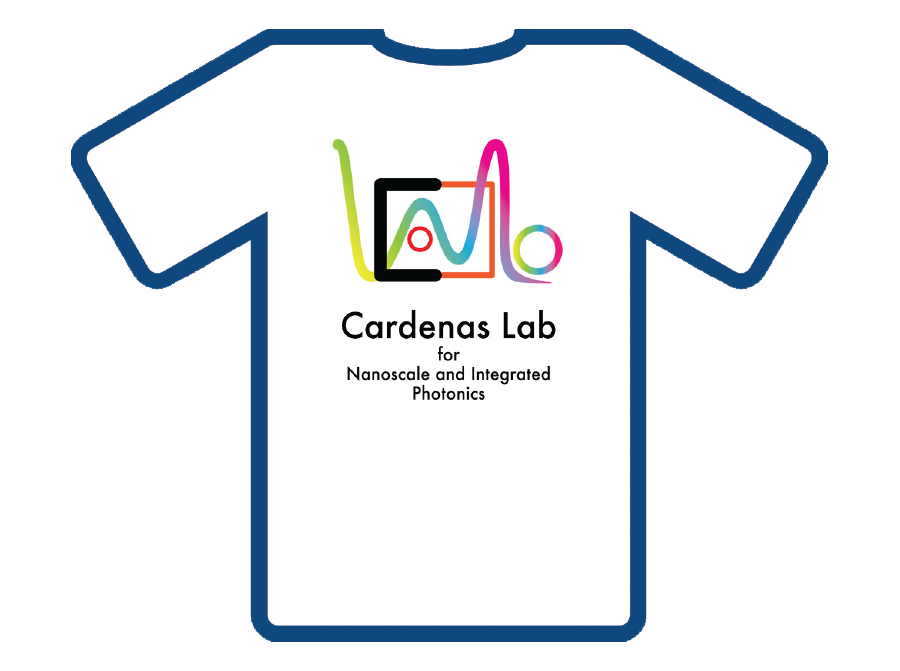
Únete a Nuestro Equipo
Si ya eres estudiante de la Universidad de Rochester encontrarás más información (en inglés) aquí.
Para entrar a estudiar en el Instituto de Óptica, sigue las instrucciones (en inglés) para solicitud de ingreso que se proporcionan en el enlace de abajo.